Approach
Our approach to working in watersheds and rivers is underpinned by the following first principles:
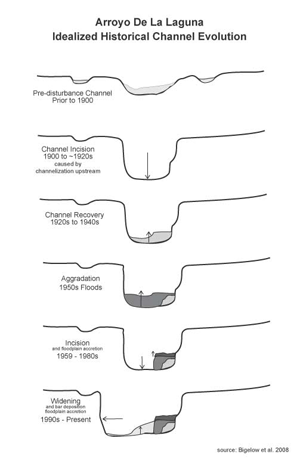
Understanding of process is more important than description of form. If flow and sediment load change, the channel form will change. Restoration of a reach, floodplain or valley floor requires working at the watershed scale to understand how upstream processes have been altered. Accordingly, restoration should focus on the root causes of the degradation, restoring processes rather than form. Similarly, historical analyses that synthesize and decipher changes in processes over time (e.g. disturbance history and regimes, annual and storm hydrographs, geomorphic and riparian controls on aquatic habitat) are more useful than anecdotal descriptions of past channel form and flow.
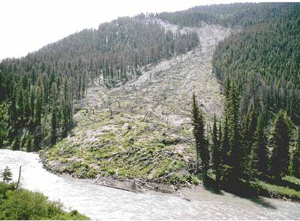
Watersheds and channels are fundamentally variable and dynamic. Channels change, stasis or inherently stable channel forms have never existed. Extreme events are often more important than average conditions. Disturbance at a variety of spatial and temporal scales is a natural and often essential component of many communities. Ultimately, the restoration of disturbance regimes may be critical for habitat and species recovery. Some terrains and climates are more stable than others, and within a watershed, downstream transport and storage segments are more stable than headwater source areas. Location is critical.
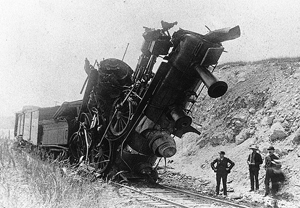
Avoid train wrecks by constructing solvable questions and working at the watershed scale. These are questions that can be answered within the precision and certainty of the science. Predicting effects of altered hydrology and sediment transport are crude (±100%) due to the stochastic nature of sediment supply, transport, and resulting channel form. Punctuated sediment supply to streams by mass wasting and bank erosion creates a high degree of spatial and temporal variability in sediment transport. Sediment storage in bars, floodplains, terraces, and behind log jams creates lag times of years to decades that complicates sediment tracking through the river network. Consequently, many questions and studies to answer them are best suited for large-scale interactions of watershed processes (i.e. working at the basin scale), where general patterns are more important than details.
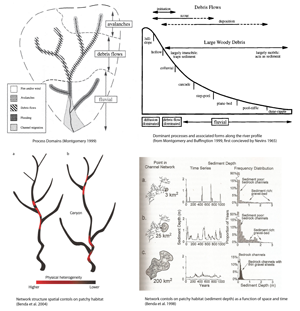
Conceptual frameworks are necessary to simplify and clarify where processes dominate and how they vary in space and time. Linear conceptual frameworks define domains within a watershed (hillslopes, hollows, channels, floodplains) characterized by dominant geomorphic processes and associated fluvial form and habitat (e.g. process domains). Where as network frameworks use channel network structure and natural disturbance to understand the spatial and temporal variations in sediment movement and aquatic environments. Here, changes in watershed structure (alternating canyons and floodplains, tributary confluences, and landslides) create discontinuity of habitats in space (patchy mosaic), while natural disturbance (storms, fires, and floods) creates discontinuities in habitat over time. Both types of frameworks are useful to simplify and understand complex watershed processes.
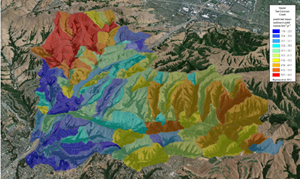
These first principles are derived primarily from the following papers:
Benda, L. 2003. The dynamic world of mountain drainage basins. A conference on water quality monitoring: spatial and temporal variability in forest water quality monitoring. December 1-2. Redding, CA.